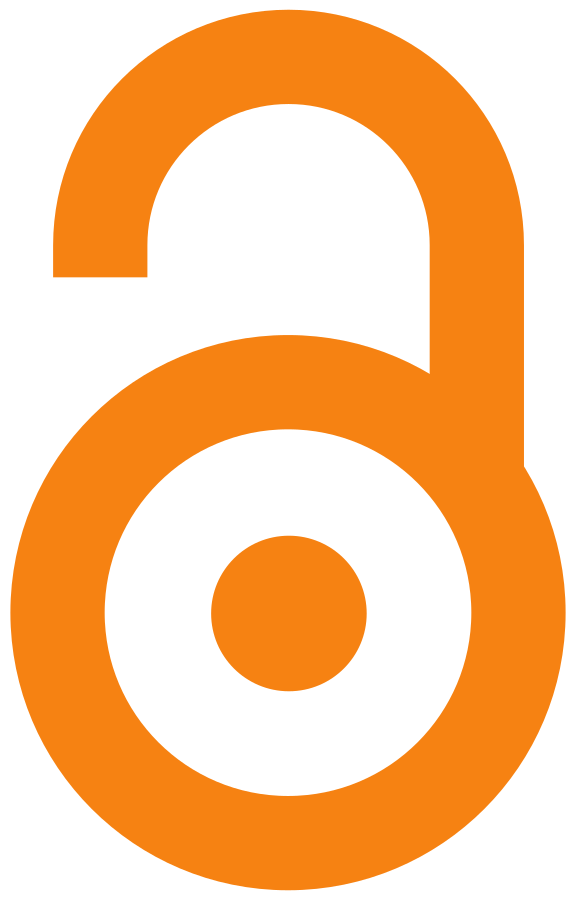
SAFE REMOTE DRUG DELIVERY USING ELECTROMAGNETIC LAUNCHERS: A FEASIBILITY STUDY
Abstract
This study investigates the feasibility and safety of using a controlled electromagnetic launcher (EML) for remote drug delivery, focusing on its potential to deliver pharmaceutical agents to targeted areas without the need for invasive procedures. The traditional methods of drug administration, such as injections or oral tablets, often present challenges, including the risk of infection, discomfort, and inefficient targeting. A controlled electromagnetic launcher offers a novel, non-invasive alternative for delivering drugs, particularly in hard-to-reach areas. The research involves the design and development of an EML system, followed by a series of tests to evaluate its accuracy, safety, and efficiency in drug delivery. The results demonstrate that the EML is capable of delivering drugs to specific locations safely, with promising implications for future medical applications.
Keywords
Electromagnetic Launcher, Remote Drug Delivery, Controlled Drug Delivery
References
Barrett-Lennard, L.; Smith, T.G.; Ellis, G.M. A cetacean biopsy system using lightweight pneumatic darts, and its effect on the behavior of killer whales. Mar. Mammal Sci. 1996, 12, 14–27. [Google Scholar] [CrossRef]
Bush, M. Remote drug delivery systems. J. Zoo Wildl. Med. 1992, 1992, 159–180. [Google Scholar]
Vekselman, V.; Sande, L.; Kornev, K.G. Fully magnetic printing by generation of magnetic droplets on demand with a coilgun. J. Appl. Phys. 2015, 118, 224902. [Google Scholar] [CrossRef]
Kreeger, T.J. Overview of delivery systems for the administration of contraceptives to wildlife. Contracept. Wildl. Manag. 1997, 1997, 29–48. [Google Scholar]
Isaza, R. Remote drug delivery. Zoo Anim. Wildl. Immobil. Anesth. 2014, 2014, 155–169. [Google Scholar]
Cattet, M.R.; Bourque, A.; Elkin, B.T.; Powley, K.D.; Dahlstrom, D.B.; Caulkett, N.A. Evaluation of the potential for injury with remote drug-delivery systems. Wildl. Soc. Bull. 2006, 34, 741–749. [Google Scholar] [CrossRef]
Hairgrove, T.; Gill, R.; Waters, C.; Miller, R.; Mays, T.; Miller, M.; Fajt, V. Does dart gun delivery of antibiotics cause changes in drug disposition or meat quality? Am. Assoc. Bov. Pract. Conf. Proc. 2016, 2016, 167–168. [Google Scholar] [CrossRef]
Pagano, A.M.; Peacock, E.; McKinney, M.A. Remote biopsy darting and marking of polar bears. Mar. Mammal Sci. 2014, 30, 169–183. [Google Scholar] [CrossRef]
Noren, D.P.; Mocklin, J.A. Review of cetacean biopsy techniques: Factors contributing to successful sample collection and physiological and behavioral impacts. Mar. Mammal Sci. 2012, 28, 154–199. [Google Scholar] [CrossRef]
Bearzi, G. First report of a common dolphin (Delphinus delphis) death following penetration of a biopsy dart. J. Cetacean Res. Manag. 2000, 2, 217–222. [Google Scholar] [CrossRef]
Tarmizi, M.R.; Zainuddin, Z.Z. Low-cost remote drug delivery blow-dart for veterinary use. J. Wildl. Parks 2020, 35, 39–48. [Google Scholar]
Economidou, S.; Uddin, M.; Marques, M.; Douroumis, D.; Sow, W.; Li, H.; Reid, A.; Windmill, J.; Podoleanu, A. A novel 3D printed hollow microneedle microelectromechanical system for controlled, personalized transdermal drug delivery. Addit. Manuf. 2021, 38, 101815. [Google Scholar] [CrossRef]
Philips, B. How Accurate Is a 300fps Nerf Blaster? 30 May 2020. Available online: https://www.youtube.com/watch?v=vZtZMJEb9k4 (accessed on 1 June 2022).
Gallagher, G.R.; Petzinger, C.; Rieger, J.A.; Dziurzynski, A.D.; Thayer, O. Development of a Safer Tranquilizer Dart. Proc. Vertebr. Pest Conf. 2010, 24, 1. [Google Scholar] [CrossRef]
Horst, D.J. 3D printing of pharmaceutical drug delivery systems. Arch. Org. Inorg. Chem. Sci. 2018, 1, 1–5. [Google Scholar] [CrossRef]
Jain, A.; Bansal, K.K.; Tiwari, A.; Rosling, A.; Rosenholm, J.M. Role of polymers in 3D printing technology for drug delivery-an overview. Curr. Pharm. Des. 2018, 24, 4979–4990. [Google Scholar] [CrossRef] [PubMed]
Lui, Y.S.; Sow, W.T.; Tan, L.P.; Wu, Y.; Lai, Y.; Li, H. 4D Printing and Stimuli-responsive Materials in Biomedical Applications. Acta Biomater. 2019, 92, 19–36. [Google Scholar] [CrossRef]
Martinez, P.R.; Goyanes, A.; Basit, A.W.; Gaisford, S. Fabrication of Drug-Loaded Hydrogels with Stereolithographic 3D Printing. Int. J. Pharm. 2017, 532, 313–317. [Google Scholar] [CrossRef] [PubMed]
Devi, L.; Gaba, P.; Chopra, H. Tailormade Drug Delivery System: A Novel Trio Concept of 3DP+ Hydrogel+ SLA. J. Drug Deliv. Ther. 2019, 9, 861–866. [Google Scholar] [CrossRef]
Trenfield, S.; Awad, A.; Madla, C.; Hatton, G.; Firth, J.; Goyanes, A.; Gaisford, S.; Basit, A. Shaping the future: Recent advances of 3D printing in drug delivery and healthcare. Expert Opin. Drug Deliv. 2019, 16, 1081–1094. [Google Scholar] [CrossRef]
Massei, G.; Cowan, D. Fertility control to mitigate human–wildlife conflicts: A review. Wildl. Res. 2014, 41, 1–21. [Google Scholar] [CrossRef]
Allen, L.; Ansel, H.C. Ansel’s Pharmaceutical Dosage Forms and Drug Delivery Systems; Lippincott Williams & Wilkins: Philadelphia, PN, USA, 2013. [Google Scholar]
Lepowsky, E.; Tasoglu, S. 3D printing for drug manufacturing: A perspective on the future of pharmaceuticals. Int. J. Bioprinting 2018, 4, 1. [Google Scholar] [CrossRef]
Xing, J.F.; Zheng, M.L.; Duan, X.M. Two-photon polymerization microfabrication of hydrogels: An advanced 3D printing technology for tissue engineering and drug delivery. Chem. Soc. Rev. 2015, 44, 5031–5039. [Google Scholar] [CrossRef] [PubMed]
Cho, H.; Jammalamadaka, U.; Tappa, K. Nanogels for pharmaceutical and biomedical applications and their fabrication using 3D printing technologies. Materials 2018, 11, 302. [Google Scholar] [CrossRef]
Mobaraki, M.; Ghaffari, M.; Yazdanpanah, A.; Luo, Y.; Mills, D.K. Bioinks and bioprinting: A focused review. Bioprinting 2020, 18, e00080. [Google Scholar] [CrossRef]
Rosenfield, D.A.; Acosta, A.; Tavares, D.T.; Pizzutto, C.S. Potential remote drug delivery failures due to temperature dependent viscosity and drug-loss of aqueous and emulsion-based fluids. J. Threat. Taxa 2021, 13, 17639–17645. [Google Scholar] [CrossRef]
Walcott, H.E.; Curanovic, T.; Ghani, B.S.E.N. The Development and Testing of a Dual Function Underwater Dart Rifle. 2012. Available online: https://www.researchgate.net/profile/Horace-Walcott/publication/344179992_2012_PROCEEDINGS_AAZV_CONFERENCE_257_THE_DEVELOPMENT_AND_TESTING_OF_A_DUAL_FUNCTION_UNDERWATER_DART_RIFLE/links/5f5993e94585154dbbc3fec0/2012-PROCEEDINGS-AAZV-CONFERENCE-257-THE-DEV (accessed on 1 June 2022).
Rosenfield, D.; Ferraro, M.; Igayara, C.; Cortopassi, S.R.G.; Pizzutto, C.S. Chemical immobilization of free-living capybaras (Hydrochoerus hydrochaeris) using ketamine-dexmedetomidine combination and a remote drug delivery system. Braz. J. Vet. Med. 2020, 42, e107220. [Google Scholar]
Wolfe, L.L.; Miller, M.W. Using tailored tranquilizer combinations to reduce stress associated with large ungulate capture and translocation. J. Wildl. Dis. 2016, 52, S118–S124. [Google Scholar] [CrossRef]
Article Statistics
Downloads
Copyright License
Copyright (c) 2025 Daniel R. Harris (Author)

This work is licensed under a Creative Commons Attribution 4.0 International License.
Authors retain the copyright of their manuscripts, and all Open Access articles are disseminated under the terms of the Creative Commons Attribution License 4.0 (CC-BY), which licenses unrestricted use, distribution, and reproduction in any medium, provided that the original work is appropriately cited. The use of general descriptive names, trade names, trademarks, and so forth in this publication, even if not specifically identified, does not imply that these names are not protected by the relevant laws and regulations.